1. Analysis of materials and functions related to clean energy utilization
The development and utilization of clean energy to replace fossil fuels is a major challenge of this century. Clean energy needs to be used, stored, and transported, which requires the development of new materials as well as new devices with high functionality and durability. We analyze the structures and functions of materials and devices from the design of the analysis equipment, and feed back the obtained results to the development. In addition to the experiments in laboratory, we sometimes use large-scale experimental facilities such as synchrotron radiation X-rays and neutron beams.
(a) Analysis of clean energy materials
For understanding clean energy materials constructed from the atomic level to millimeters and centimeters, hierarchical analysis is required. Therefore, it is necessary to combine various analysis methods.
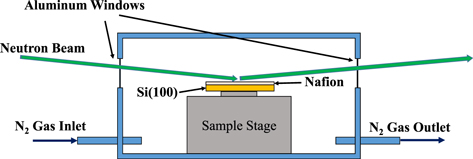
Here, as an example, the results of neutron beam reflectance measurements performed at the Materials and Life Science Experimental Facility (MLF) of the Japan Proton Accelerator Research Complex (J-PARC) are shown. A polymer electrolyte fuel cell (PEFC) uses a polymer electrolyte membrane with a thickness of about 10 μm that allows hydrogen ions to pass through but does not allow electrons to pass through. Normally, the required polymer is dissolved in a solvent, poured (cast) onto a flat glass plate, dried, and incorporated into the PEFC. After a thin film formed by casting is incorporated into a fuel cell in this way, it is difficult to achieve sufficient performance. It is normal to first rub the surfaces of the thin film with a special method or to generate electricity for a while before finally the cell performance is demonstrated.
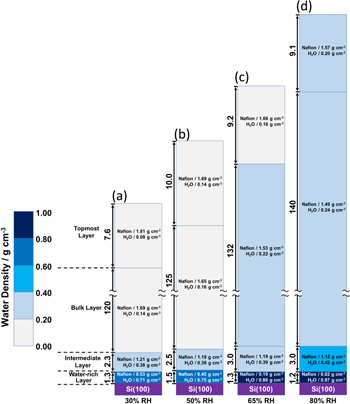
In order to investigate the reason for this, we fabricated an electrolyte film, Nafion, with a thickness of about 0.1 μm on a flat silicon oxide (model of a glass plate) at the nanometer level, and installed it in a device for the neutron reflectance shown in the figure above. In this way, the layered structure of Nafion thin films was measured under a humidified nitrogen atmosphere.
The figure below shows the results when the temperature was 80°C and the relative humidity was varied from 30% to 80%. As a result, the Nafion membrane was found to have a four-layered structure, and each layer swelled at a different rate. In particular, it was found that the surface in contact with silicon oxide (the surface in contact with glass) was highly hydrophilic, whereas the surface in contact with nitrogen was hydrophobic. These experiments clarified the properties of Nafion immediately after film formation, and are expected to contribute to improved performance.
(b) Analysis of clean energy devices
After developing a new material or device with the new materials used, understanding the internal state of the device during operation provides feedback for performance improvement.
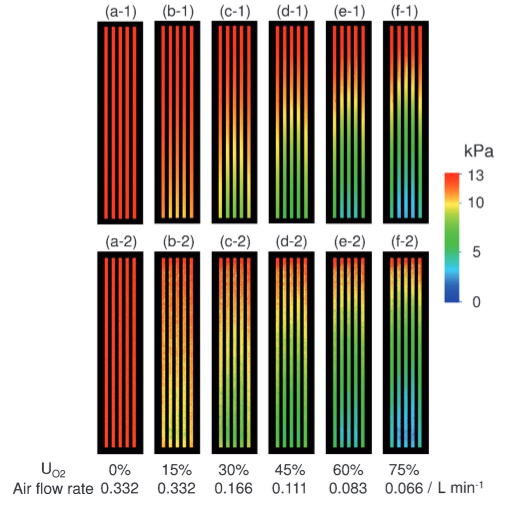
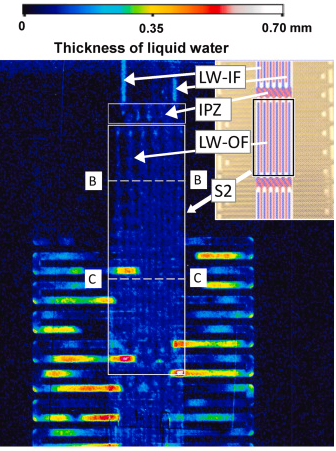
The figure on the left is a visualization image of the partial pressure of oxygen inside the PEFC during power generation using a special dye. Clearly seen is how oxygen is consumed downwards in the straight channels. Differences in oxygen partial pressure can be observed channel by channel with a resolution of 1 μm. The figure on the right is a visualized image of water accumulated inside a newly-structured PEFC using J-PARC neutron beams. The relationship between water distribution and power generation performance was understood for the first time.
By incorporating these measurement results into the reaction-analysis software of the fuel cell, the distribution of parameters, such as hydrogen, steam, current density, and temperature, can be understood, and can be used to develop higher performance materials and devices.
2. Measurement techniques related to ionization methods and ion beams
We live surrounded by a variety of materials. We take oxygen from the gaseous air and eat liquid and solid foods, and they are converted into energy or stored in our bodies. The material exists mainly in gaseous, liquid or solid form and is mostly electrically neutral, but some materials exist with electric charges. When a material is positively or negatively charged, it is called “ion” and the process of bringing it to the state is called “ionization”. This division focuses on ionization methods and ion beams, and the measuremet techniques related to them are studied.
(a) Research on ionization methods for mass spectrometry
If you want to know the weight of something around you, you can measure it using a bathroom scale or a simple balance. However, the weight of extremely small materials at the atomic or molecular level cannot be measured with these methods. The method to measure the mass of a single atom or molecule is called “mass spectrometry”. Note that “mass” is a universal quantity that does not change anywhere in the universe, but weight is the magnitude of the gravitational force acting on the material, so it changes depending on where the material exists. Mass spectrometry also cannot directly measure the mass of material. In mass spectrometry, a material is first ionized by a suitable method and then transported in the electric or magnetic fields, and then its mass is measured by observing its motion. As the material is observed in its ionic state, the quantity measured by mass spectrometry is not the mass (m) itself, but the ratio (m/z) of the mass to the number of charges (z) of the ion. The “m/z” is called the mass-to-charge ratio or m over z. In mass spectrometry, m is expressed in the unified atomic mass (u), defined as 1/12 of the mass of one carbon atom (12C) with mass number 12. If the charge number z of an ion is 1, the value obtained by mass spectrometry itself corresponds to the mass of the ion (unit u), but the charge number is not always 1.Ionizing a material is essential for mass spectrometry. Therefore, the performance of mass spectrometry depends strongly on the efficiency of ionization. An ionization method is appropriately selected according to whether the material to be analyzed is in a gaseous, liquid, or solid state. Many ionization methods have been studied and developed for gases, liquids and solids. A wide variety of ionization methods are available, for example, using the discharge phenomenon for gases, the electrospray phenomenon for liquids, and irradiation of lasers or ion beams (see next section) for solids. This division conducts research on unique ionization methods and ion sources suitable for gases, liquids, and solids respectively. As research examples, a robotic instrument for automated sampling and consecutive mass spectrometry (left figure) and a mass spectrometry system combining heat pulse desorption and proximity corona discharge ionization (right figure) are shown.
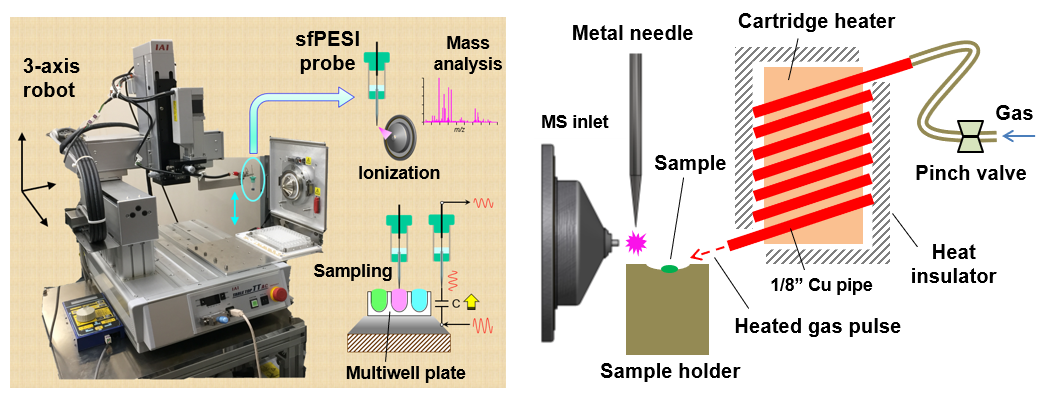
What are the advantages of measurement of the mass of material by mass spectrometry? Our bodies are made up of various molecules such as proteins and lipids, but if, for example, the cells in the bodies produce molecules that are different from the normal states due to some disease, the mesurement of these molecules by mass spectrometry can lead to the early detection of the disease and the investigation of the cause of the disease. Dangerous materials to society such as explosives or narcotics may be brought into places where many people gather. If dangerous materials are detected rapidly with high sensitivity by mass spectrometry, various hazards can be eliminated, leading to a safe and secure society. In this division, research is carried out to ensure the effective use of mass spectrometry in medicine and social life.
(b) Research on new ion beams for surface analysis
When materials are positively or negatively ionized and grouped into a line, it is called an “ion beam”. When an ion beam is irradiated onto a solid, the solid is modified by the energies of the ion beam, or constituents are ejected from the surface of the solid. If the various phenomena caused by ion beam irradiation are utilized effectively, it is possible to etch very small areas of a solid (microfabrication), change the electrical properties (ion implantation), remove small particles from the surface of a solid (surface cleaning), attack and treat cancer cells deep within the human body (particle beam cancer therapy). When an ion beam is irradiated onto a solid, the electrons (secondary electrons) and ions (secondary ions) emitted from its surface can be detected to investigate the properties of the solid. This means that ion beams can be used as measurement techniques. Secondary ion mass spectrometry (SIMS) is a measurement technique for analyzing the mass-to-charge ratio (m/z) of secondary ions generated by ion beam irradiation. In SIMS, ion beams of atoms and molecules (atomic and molecular ion beams), the smallest units of materials, were initially used, and in recent years, aggregations of atoms and molecules (cluster ion beams) have also been used as ion beams. Cluster ion beams of the polyatomic molecule fullerene (C60) and metal clusters (gold and bismuth) have been commercialized and used as ion beams in state-of-the-art SIMS instruments.
In this division, research is carried out to create new ion beams to further improve the performance of surface analysis instruments using ion beams such as SIMS and X-ray photoelectron spectroscopy. We focus on the electrospray phenomenon, which is frequently used as an ionization method for liquids in mass spectrometry, and would like to utilize the charged micro-droplets (droplet ions) generated by the electrospray in vacuum as ion beams. When the droplet ions are generated at atmospheric pressure, the droplet ions diffuse as shown in the upper left figure (deceleration by collision with the atmosphere and Coulomb repulsion between droplet ions), but when generated under vacuum, the straight droplet ion beams can be obtained as shown in the upper right figure. This should be because the Coulomb repulsion effect becomes negligible for the droplet ions accelerated in vacuum. This means that vacuum electrospray can produce droplet ion beams with high intensity and brightness.
The lower left figure shows a vacuum electrospray droplet ion (V-EDI) beam generator. The V-EDI beam generator was installed in a SIMS instrument and extremely high secondary ion yields without fragmentation for several biomolecules were obtained. This leads to a significant improvement in the analytical performance of SIMS. The aim is to put this droplet ion beam into practical use as a new measurement technique for surface analysis instruments, as shown in the lower right figure.
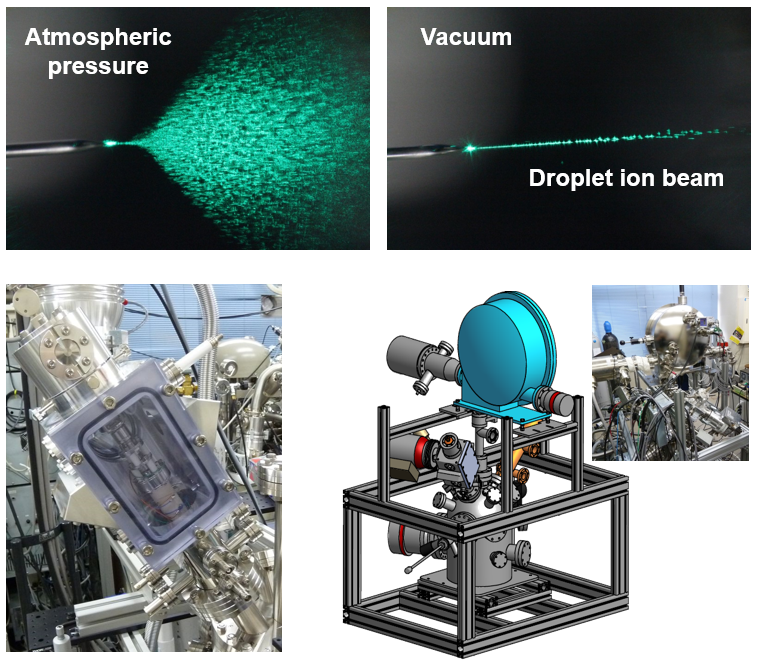